Squidsat: America's Next Great Cubesat
- Jonathan Durkin
- Mar 10
- 4 min read
Systems Engineering at the Frontier of CubeSat Development
Within the rapidly evolving landscape of small satellite technology, Arizona State University's Interplanetary Laboratory continues to build upon its established legacy of innovation. Following the successful missions of Phoenix, DORA, and Coconut, the laboratory's latest endeavor—SquidSat—represents the next evolution in ASU's CubeSat development program. As one of the few graduate students involved in this primarily undergraduate initiative, my role as Systems Engineer and Project Manager offers a unique challenge on both the technical and leadership dimensions of spacecraft development.
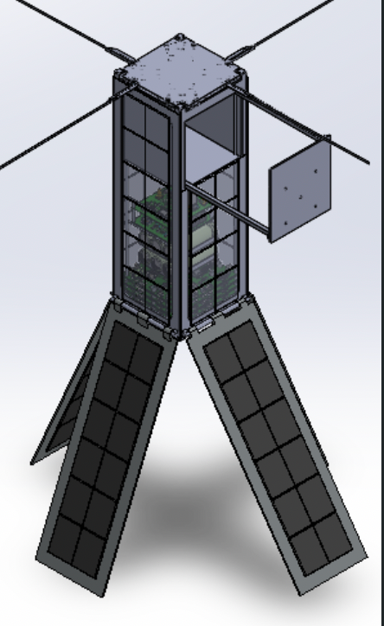
From Proposal to Participation
My journey to the SquidSat team followed an unexpected path. Initially, I had developed an independent CubeSat proposal—ODDSTAR—which, while not ultimately selected for development, demonstrated sufficient technical merit to earn me an invitation to join the SquidSat mission. This transition from conceptual design to systems engineering has provided valuable insights into the iterative nature of space mission development and the importance of adaptability in technical careers.
The role of Systems Engineer in a small satellite mission serves as a nexus point where technical domains intersect with project management requirements. My primary responsibility involves ensuring that subsystem decisions remain harmonious with overall mission objectives—preventing the technical equivalent of the right hand working against the left. This position requires maintaining both a comprehensive view of mission architecture and sufficient technical depth to evaluate subsystem integration challenges.
Dual-Payload Innovation
What distinguishes SquidSat technically is its innovative dual-payload architecture, each representing different frontiers in space technology development:
The primary payload features a programmable metasurface array—a novel microelectronic structure that functions as a customizable, programmable antenna system. This technology, developed in collaboration with ASU's Luminosity Lab, has potential applications in satellite-to-satellite power beaming and advanced communications. The in-orbit validation of this technology could significantly impact future small satellite capabilities, potentially enabling more efficient power distribution and communication between spacecraft in distributed mission architectures. But wait, what the hell is a programmable metasurface. Well compared to a traditional antenna or array, where the entire thing is controlled by a single switch on the output for example, imagine an array of micro electronic antennas or little squares with their own switches. This is done by prefabrication and careful design and is possible due to advancements in material science.

The secondary payload consists of a semiconductor device undergoing space environment testing to evaluate its durability and performance characteristics in the harsh conditions beyond Earth's protective atmosphere. This testing represents a critical step in qualifying new electronic components for future space missions, where radiation effects and extreme temperature fluctuations can dramatically impact component reliability.
These payloads are integrated into a 3U CubeSat bus—approximately the size of a shoebox—complete with deployable solar arrays and a communications system for transmitting experimental data back to Earth. The compact form factor presents significant design challenges, requiring careful consideration of power budgets, thermal management, and mechanical interfaces.
Current Focus: Payload User Guides
This semester, my work centers on developing comprehensive payload user guides for both experimental systems. These documents serve as critical interfaces between payload developers and the spacecraft bus team, defining essential parameters including:
Power requirements and profiles
Data interfaces and formats
Command structures
Thermal constraints
Physical integration specifications
Testing procedures
The development of these guides requires cross-disciplinary collaboration with both the Luminosity Lab for the metasurface array and the semiconductor research team. This collaboration exemplifies the interdisciplinary nature of space systems engineering, where effective communication across technical domains becomes as important as the technical work itself.
Academic Integration and Skill Development
What makes this project particularly valuable from an educational perspective is its direct integration with my graduate program in Space Systems Design and Engineering. The hands-on experience complements my coursework, providing practical application of systems engineering principles in a real mission context.
To build the necessary technical foundation for this role, I'm simultaneously pursuing SciTech Leadership courses covering project management, space finance, and team leadership, alongside a Harvard online program focused on AI/ML applications using Python. These formal educational components provide structured knowledge that I can immediately apply within the SquidSat development process.
The technical skills being developed through this project span multiple domains, including:
Arduino programming and embedded systems
Electronic component soldering and assembly
Additive manufacturing techniques
Basic electrical engineering principles
Software development and integration
Systems engineering methodologies
This multidisciplinary skill development exemplifies the modern approach to space systems education, where breadth of knowledge becomes as crucial as depth in any single domain. You know, a lot of the weaknesses I have in terms of technical skill, coming from more of a science background with my Astrobiology and entrepreneurship experience. End goal: build the skills to design missions and become a PI.
Collaborative Framework
The organizational structure supporting SquidSat highlights ASU's approach to space technology development. The project operates at the intersection of three key university entities:
The Sun Devil Satellite Laboratory, providing student-led spacecraft development expertise
The Interplanetary Laboratory, offering technical guidance and mission heritage
The Luminosity Lab, contributing innovative payload technology
This collaborative framework creates a unique educational ecosystem where students gain exposure to different organizational cultures and technical approaches—mirroring the complex stakeholder environments of professional space missions.
Looking Forward
As SquidSat progresses through its development cycle, it will likely evolve into a cornerstone of my master's program. The systems engineering challenges encountered and resolved through this process will provide both technical content and methodological insights for potential thesis work. More importantly, this project represents the practical application of space systems theory in a tangible engineering challenge. Rather than remaining in the realm of hypothetical design exercises, SquidSat demands real-world solutions to the complex problems of spacecraft development—from thermal management to communications link budgets, from power generation to attitude control.
While challenging, this opportunity to contribute to ASU's small satellite legacy offers invaluable professional development that no classroom experience alone could provide. As SquidSat moves from concept to orbital deployment, it will carry not only its experimental payloads but also the educational journey of every student involved in its creation.
This project is ongoing with the Sun Devil Satellite Lab, the Interplanetary Lab, and the Luminosity Lab at Arizona State University's School of Earth and Space Exploration. Future updates will document key development milestones and technical challenges encountered throughout the mission lifecycle.
コメント